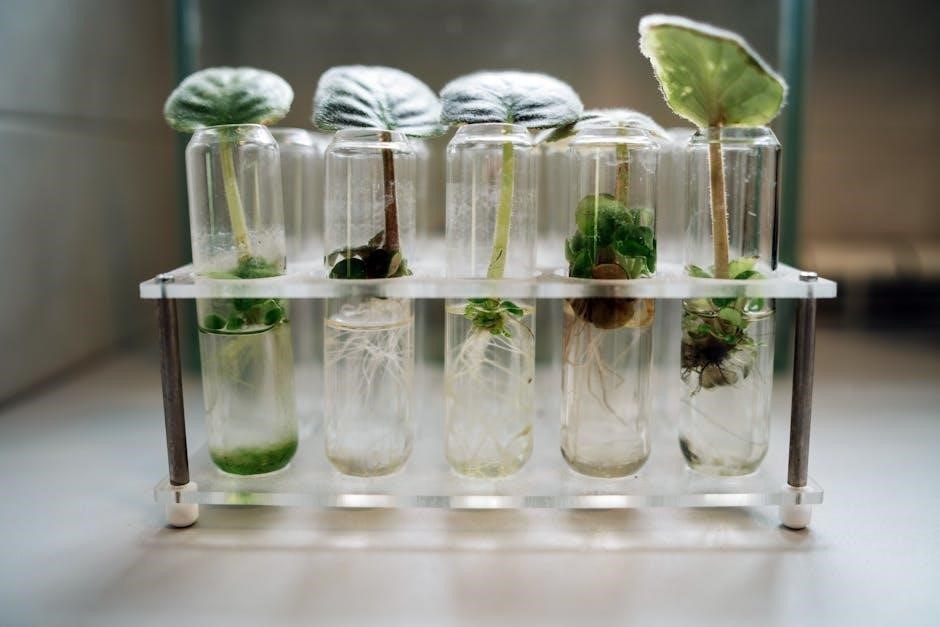
study guide photosynthesis
Photosynthesis is the essential biological process where light energy
converts into chemical energy‚ fueling life on Earth. This remarkable
process sustains ecosystems by providing energy and releasing oxygen into
the atmosphere.
Definition of Photosynthesis
Photosynthesis‚ at its core‚ is the biochemical pathway employed by
photoautotrophs to transform solar energy into usable chemical energy‚
primarily in the form of glucose. This intricate process harnesses light‚
water‚ and carbon dioxide to synthesize carbohydrates‚ simultaneously
releasing oxygen as a byproduct. It exclusively occurs within chloroplasts‚
organelles containing photosynthetic pigments like chlorophyll a‚ chlorophyll
b‚ carotene‚ and xanthophyll‚ vital for capturing light energy. Through
photosynthesis‚ plants and other autotrophic organisms synthesize their
nutrients‚ ensuring their survival and supporting the broader ecosystem.
Essentially‚ photosynthesis underpins the food chain‚ providing the
foundation for life as we know it.
Importance of Photosynthesis on Earth
Photosynthesis holds paramount importance for life on Earth‚ acting as the
primary mechanism for converting light energy into chemical energy that
sustains ecosystems. It is indispensable for the survival of green plants‚
algae‚ and certain bacteria‚ which form the base of most food chains.
Without photosynthesis‚ there would be no green plants‚ consequently
disrupting the entire food web and impacting animal life. Furthermore‚
photosynthesis plays a critical role in maintaining atmospheric balance by
absorbing carbon dioxide‚ a greenhouse gas‚ and releasing oxygen‚ essential
for respiration in many organisms. This process helps regulate Earth’s
climate and supports a habitable environment for diverse life forms.
The Photosynthesis Process
The photosynthesis process involves converting light energy into chemical
energy‚ using carbon dioxide and water. This complex process creates glucose
and releases oxygen‚ essential for life on Earth’s ecosystems.
Overall Photosynthesis Equation
The overall photosynthesis equation succinctly summarizes the entire process‚
illustrating how plants convert light energy into chemical energy. It
demonstrates the transformation of carbon dioxide and water into glucose and
oxygen‚ essential for life. The balanced equation highlights the precise
quantities of each molecule involved‚ showcasing nature’s elegant stoichiometry.
Specifically‚ 6 molecules of carbon dioxide plus 6 molecules of water‚ in
the presence of light energy‚ yield one molecule of glucose and 6 molecules
of oxygen. This equation underscores the fundamental role of photosynthesis
in producing the oxygen that supports aerobic life and the glucose that
serves as a primary energy source for many organisms. Understanding the
photosynthesis equation is crucial for comprehending the foundations of
biological energy transfer.
Reactants and Products of Photosynthesis
Photosynthesis involves specific reactants that are essential for the
process to occur‚ along with distinct products that are generated as a
result. The primary reactants are carbon dioxide‚ which plants obtain from
the air‚ and water‚ absorbed from the soil. Light energy‚ captured by
chlorophyll‚ serves as the driving force. These reactants undergo a series
of complex reactions within the chloroplasts of plant cells.
The main products of photosynthesis are glucose‚ a sugar molecule that
provides energy for the plant‚ and oxygen‚ which is released into the
atmosphere. Glucose is utilized by the plant for growth‚ development‚ and
various metabolic processes. Oxygen is a vital byproduct that supports the
respiration of most living organisms.
Steps of Photosynthesis
Photosynthesis occurs through two main stages. First‚ light-dependent
reactions capture light energy. Then‚ light-independent reactions (Calvin
Cycle) use this energy to produce glucose. Each step is crucial for
converting light into chemical energy.
Light-Dependent Reactions (Light Reactions)
The light-dependent reactions‚ the initial phase of photosynthesis‚ occur in
the thylakoid membranes of chloroplasts. This stage harnesses light energy‚
converting it into chemical energy in the form of ATP and NADPH.
Chlorophyll‚ a pigment within the thylakoids‚ absorbs light‚ initiating a
series of electron transfers.
Water molecules are split‚ releasing oxygen as a byproduct and providing
electrons to replenish chlorophyll. These energized electrons move through
an electron transport chain‚ generating a proton gradient that drives ATP
synthesis via chemiosmosis. Simultaneously‚ NADP+ is reduced to NADPH‚
another energy-carrying molecule. The ATP and NADPH produced during these
reactions are essential for the subsequent light-independent reactions‚
powering the synthesis of glucose from carbon dioxide.
Light-Independent Reactions (Calvin Cycle)
The light-independent reactions‚ also known as the Calvin cycle‚ take place
in the stroma of the chloroplast. This cycle utilizes the ATP and NADPH
generated during the light-dependent reactions to fix carbon dioxide and
produce glucose. The process begins with carbon fixation‚ where carbon
dioxide combines with ribulose-1‚5-bisphosphate (RuBP)‚ a five-carbon
molecule.
This unstable six-carbon compound immediately splits into two molecules of
3-phosphoglycerate (3-PGA). ATP and NADPH then convert 3-PGA into
glyceraldehyde-3-phosphate (G3P)‚ a three-carbon sugar. Some G3P is used
to synthesize glucose‚ while the remaining G3P is used to regenerate RuBP‚
ensuring the cycle can continue. The Calvin cycle represents the crucial
step where inorganic carbon is transformed into organic molecules‚ providing
the foundation for plant growth and energy storage.
Factors Affecting Photosynthesis
Photosynthesis rates are influenced by various environmental factors‚ including
light intensity‚ carbon dioxide concentration‚ water availability‚ and
temperature. Optimal conditions for these factors lead to maximized
photosynthetic output.
Light Intensity
Light intensity plays a crucial role in photosynthesis‚ as it directly impacts
the rate at which light-dependent reactions occur. As light intensity
increases‚ the rate of photosynthesis generally increases up to a certain
point. This is because more light energy is available to excite chlorophyll
molecules‚ leading to a greater production of ATP and NADPH‚ which are
essential for the Calvin cycle.
However‚ there is a saturation point beyond which increasing light intensity
no longer enhances photosynthesis. In fact‚ excessively high light levels can
damage photosynthetic pigments and enzymes‚ leading to a decrease in
photosynthetic efficiency. The specific light intensity required for optimal
photosynthesis varies depending on the plant species and its adaptation to
different light environments. Plants adapted to shady conditions often have
lower saturation points compared to plants that thrive in full sunlight.
Carbon Dioxide Concentration
Carbon dioxide (CO2) concentration is a critical factor influencing the rate
of photosynthesis‚ particularly in the light-independent reactions (Calvin
cycle). CO2 serves as the primary carbon source for the synthesis of glucose
and other organic molecules. As CO2 concentration increases‚ the rate of
carbon fixation by the enzyme RuBisCO also increases‚ leading to a higher
rate of photosynthesis.
However‚ similar to light intensity‚ there is a saturation point for CO2
concentration. Once this point is reached‚ increasing CO2 levels no longer
enhance the rate of photosynthesis. In some cases‚ excessively high CO2
concentrations can even inhibit photosynthesis by interfering with other
enzymatic reactions or causing stomatal closure‚ which limits gas exchange.
The optimal CO2 concentration for photosynthesis varies depending on the plant
species and environmental conditions.
Water Availability
Water is essential for photosynthesis‚ acting as a reactant in the light-dependent
reactions and maintaining turgor pressure for stomatal opening. Water
availability significantly impacts photosynthetic efficiency. When water is
scarce‚ plants experience water stress‚ triggering various physiological
responses to conserve water.
One of the primary responses to water stress is stomatal closure. Stomata are
small pores on the surface of leaves that allow for gas exchange‚ including
the uptake of carbon dioxide (CO2) and the release of oxygen (O2). When water
is limited‚ stomata close to reduce water loss through transpiration. However‚
this closure also restricts CO2 entry into the leaves‚ thereby limiting the
substrate availability for the Calvin cycle.
Temperature
Temperature plays a crucial role in photosynthesis as it influences the
enzymatic reactions involved in both the light-dependent and
light-independent stages. Photosynthesis relies on enzymes to catalyze various
biochemical reactions‚ and these enzymes have optimal temperature ranges for
their activity. Generally‚ photosynthetic rates increase with temperature up to
a certain point‚ beyond which they start to decline.
High temperatures can denature enzymes‚ disrupting their structure and
function‚ thus inhibiting photosynthetic activity. In contrast‚ low
temperatures can slow down the rate of enzymatic reactions‚ reducing the overall
efficiency of photosynthesis. The optimal temperature range for photosynthesis
varies among plant species‚ depending on their adaptation to different
climatic conditions.
Photosynthetic Pigments
Photosynthetic pigments‚ such as chlorophylls and carotenoids‚ are vital for
capturing light energy during photosynthesis. These pigments absorb specific
wavelengths of light‚ initiating the process of converting light into chemical
energy.
Chlorophyll a and b
Chlorophyll a and b are the primary photosynthetic pigments in plants and
algae‚ playing a crucial role in absorbing light energy to drive
photosynthesis. Chlorophyll a is directly involved in the light-dependent
reactions‚ converting light energy into chemical energy by exciting
electrons. Chlorophyll b‚ on the other hand‚ acts as an accessory pigment‚
capturing light energy and transferring it to chlorophyll a.
These pigments absorb light most efficiently in the blue-violet and red
portions of the electromagnetic spectrum‚ reflecting green light‚ which is
why plants appear green. The slight structural difference between chlorophyll
a and chlorophyll b results in slightly different absorption spectra‚ allowing
them to capture a broader range of light wavelengths and enhance the
efficiency of photosynthesis.
Carotenoids and Xanthophylls
Carotenoids and xanthophylls are accessory pigments that broaden the range of
light wavelengths captured by plants for photosynthesis. Carotenoids‚ which
include beta-carotene‚ absorb light in the blue-green region of the spectrum‚
while xanthophylls‚ such as lutein‚ absorb blue-violet light.
These pigments transfer the energy they absorb to chlorophyll‚ enhancing the
overall efficiency of photosynthesis. Beyond their role in light capture‚
carotenoids and xanthophylls also protect chlorophyll from photo-oxidative
damage. Excessive light can create reactive oxygen species that damage
chlorophyll molecules. Carotenoids and xanthophylls scavenge these harmful
molecules‚ preventing damage and maintaining the integrity of the
photosynthetic apparatus. Their presence often becomes visible in autumn when
chlorophyll breaks down‚ revealing the vibrant yellow and orange hues of
these underlying pigments.